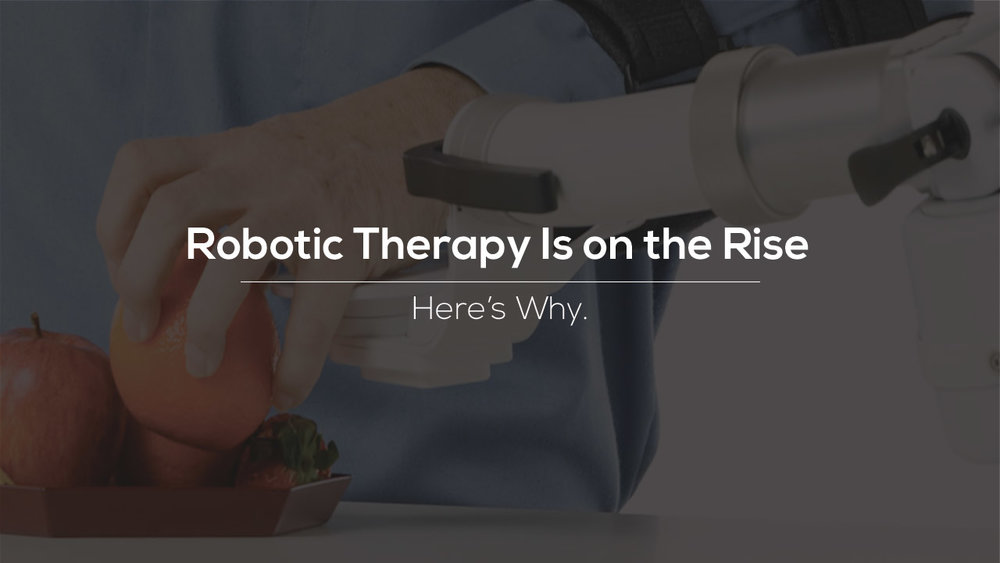
If you work in physical rehabilitation, you’ve probably noticed a major shift in thinking regarding stroke rehab.
These days, it’s generally accepted that high-intensity dosing is vital for optimum recovery following strokes. The problem is that achieving these high-dosage levels is extremely difficult using traditional techniques.
While the demand for increased dosage continues to grow, contextual and job-related demands remain the same. That makes the problem of meeting intensity demands—without increasing physical strain on therapists—a major challenge.
Active-assistive robotic therapy is changing that.
If you’re an occupational therapist, physical therapist, or physician, active-assistive robotic therapy could make a difference in your patients’ lives. Here is what you need to know about active-assistive robotic therapy, current evidence supporting its use, and the major systems on the market today.
What is active-assistive robotic therapy?
Active-assistive robotic therapy—also known as “robotic therapy,” or “robot-assisted therapy”—simply refers to the use of a robotic device in therapy.
But let’s take a step back and define what a robot actually is.
A robot is essentially a machine capable of performing complex tasks and actions—usually with an advanced level of speed and precision.
Robots vary in complexity and intelligence, and many people use them on a daily basis in their career fields. Some examples of robotic technologies might include industrial robotics in the car industry (which are designed for safer manufacturing processes), security and law enforcement-aiding robots, and robot-assisted surgical devices (including minimally invasive and remote surgery platforms).
Rehabilitation robots are their own breed.
Rehabilitation robots are intelligent devices that use sensors to monitor human movement and positioning, then use this feedback in order to interact with the environment.
These active-assistive robotic technologies are not only effective as clinical interventions, but can also help with assessments. Many of them are capable of measuring and storing parameters of patient function which can aid in long-term clinical evaluation. With the ability to detect and measure small changes in movements and forces, these devices can assist therapists in the process of treatment planning and goal setting.
What types of robotic therapy devices are out there?
Rehabilitation robotic devices fall into two categories: upper extremity (UE) and lower extremity (LE) systems.
LE rehabilitation robots include:
- Exoskeleton devices
- Foot orthoses
- In-clinic body-weight support systems, such as:
- Treadmill gait trainers
- Stationary gait trainers
- Foot-plate gait trainers
- Overground gait trainers [8]
There are two types of UE rehabilitation robots:
- Portable assistive devices or orthoses for the hand or arm
- In-clinic devices used in occupational or physical therapy
Few portable devices are available today, but many choices are available for use in the clinic.
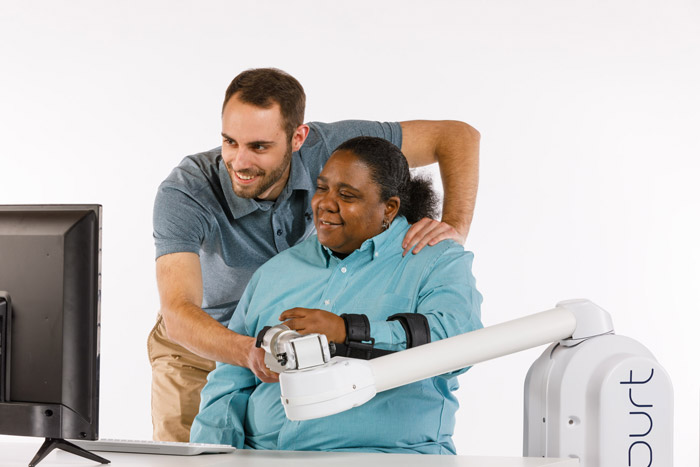
Major in-clinic upper extremity systems
There are two general types of in-clinic robotic therapy systems: exoskeletons and end-effectors.
- End-effector systems offer a faster setup and greater adaptability when compared to exoskeleton devices, which instead offer more precise joint isolation. End-effectors usually make contact with the limb at the distal end of the device, utilizing a hand rest or arm cradle to support the affected UE.
- Exoskeleton systems mirror the structure of the human skeleton and joint movements. Exoskeletons offer more precise control of joints, but they also require a longer and more complex setup time, can be a bit larger, and may feel confining for some patients.
What evidence supports robotic therapy?
New evidence shows that there’s a critical window in cortical plasticity during the first two weeks following a cerebral-vascular accident (CVA) [1].
This means that clinicians are tailoring stroke programs and interventions earlier in the rehabilitation process, and they are increasing both dosing and intensity.
Therapists are also integrating new technologies such as:
- EMG/Biofeedback
- FES (functional electrical stimulation)
- Sensor-based systems
- Virtual reality
- Brain stimulation for neurological rehabilitation
New evidence suggests that by increasing the number of motor repetitions earlier in the rehabilitation process, patients will enjoy improved UE functional recovery [1]. Active-assistive robotic therapy is a promising technology to address intensity and dosing in UE motor training.
In addition:
- Active-assistive robotic therapy has been shown to both reduce spasticity, and to improve motor function and range of motion in the upper extremity (UE) [2].
- Sensorimotor training with robotic therapy can improve motor control of the shoulder and elbow, and it can also improve upper extremity (UE) functional outcomes [3].
- Technologies such as video games or robotics can maximize motor control earlier in the rehabilitation continuum [4].
Driven by new requirements for earlier, more intensive training in stroke rehabilitation, the use of active-assistive robotic therapy is becoming increasingly common in clinical therapy settings.
How can active-assistive robotic therapy help our patients?
It provides forced use of the affected upper extremity
A review of the evidence surrounding constraint-induced movement therapy (CIMT) following stroke (such as the EXCITE trial) reveals many potential benefits of robotic therapy.
For instance, while CIMT is effective for higher-level patients with active movement in the wrist and hand, it has its limitations when used in the clinic with lower-level patients who have greater motor impairments. This has generally meant that more impaired patients miss out on the benefits of CIMT.
But one of the best applications of active-assistive robotic therapy platforms, such as the Barrett UE Robotic Trainer (BURT), is that it allows patients with trace-to-poor motor function in the upper extremity—ones who are usually considered too low-level to participate in traditional CIMT treatment—the opportunity to benefit from this type of forced-use intervention.
It creates proper intensity and dosing
Intensity is the name of the game in current clinical research. A 2012 study by John Krakauer found that animal test subjects required 400 motor repetitions per session to facilitate optimum changes in neuroplasticity following stroke [1].
But to achieve this level of high-intensity task dosing, clinicians face a dilemma: How can you meet the current standards of research and best practice—without increasing physical strain on therapists, leaving other clinical priorities unfulfilled, or breaking the rigid rules for therapy time in the clinic?
Active-assistive robotic therapies can help by providing high-dose, task-repetitive movements, while minimizing therapist fatigue and strain.
It enables gravity-eliminated planes and graded assistance
Do you ever wish that it were easier to work in gravity-eliminated planes when performing one-on-one treatments?
Upper extremity motor learning follows a natural progression—from passive to active movement, and from gravity-eliminated movements to movements against gravity. UE robots that target the shoulder let patients work in gravity-eliminated planes of the shoulder complex. Therapists can do this without compromising posture or being restricted to a tabletop surface.
Some systems even allow therapists to isolate the planes of movement associated with synergistic and reflexive movement patterns. Active robotic assistance can be graded across a spectrum, from passive range of motion (PROM) to active-assistive (AAROM) and active range of motion (AROM), individualizing each patient’s needs to maximize motor gains.
It promotes targeted and individualized treatment
Every patient is different, with different treatment needs, and occupational therapists are constantly looking for that “just right” challenge for each and every one of their patients. With active-assistive robotic therapy, you can be picky about detailed task parameters, cues, and visual stimuli, and you’ll be able to create individualized performance feedback options.
Robotic systems also offer targeted interventions to the shoulder, elbow and forearm, or hand. During robotic therapy, patients experience a sense of increased independence in the early phases of therapeutic treatment, increasing patient initiation and encouraging early volitional movement.
In traditional interventions, especially in inpatient settings, UE motor training can be limited if you don’t have a second person to assist with the treatments. Active-assistive robotic therapies help to provide a sensory-rich environment and targeted individualized treatments—without the risk of therapist fatigue.
What should we consider when purchasing robotic devices?
With several rehabilitation robotics companies in the market, UE active-assistive robotic therapy devices are becoming increasingly affordable. Here are a few factors to consider when looking at these devices.
What’s the cost?
Consider what your facility wants to invest in technology. One of the major critiques of robot-assisted therapy has been the cost; however, one analysis concluded that there was very little difference in cost between using active-assistive robotic therapy, compared to traditional intensive therapy [6]. Many rehabilitation robots have a sticker price of six figures, with exoskeletons tending to be the most expensive. In an effort to make rehabilitation robots more accessible to patients and clinics, some device manufacturers, like Barrett Technology, are coming in around $60,000.
Is the user interface engaging?
One of the major strengths of these devices is they increase patient engagement. Make sure that the games the system offers are fun and motivating for your patents.
What area do I want to target?
Devices may target the hand, wrist, elbow, shoulder complex, or a combination of these.
Does the device have the features you want?
Pay attention to the available range of motion (ROM) and anatomical movements the device features. Active-assistive robotic therapy devices may include some or all of these features:
- Active exercise
- Active robotic assistive exercise
- Passive exercise
- Gravity elimination
- Haptic features (typically vibration or resistance)
- Coaching feedback [7]
Also, ask if the device has features to combat tone/reflexive movement, and inquire about any additional cognitive features. Ask about setup time, and then ask yourself whether the time required is appropriate for your facility, given your setting and therapy time constraints.
What types of robotic rehabilitation devices are out there?
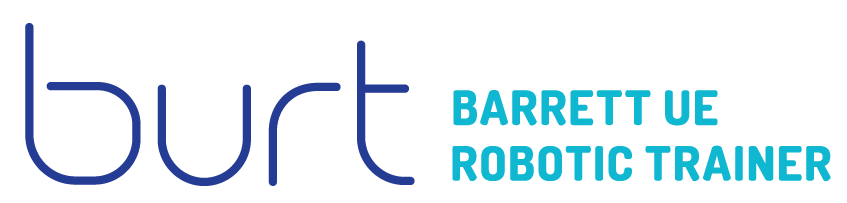
The Barrett Upper Extremity Robotic Trainer (BURT)
BURT is a rehabilitation robot for the shoulder complex and elbow that was designed based on the latest research on high-intensity repetitions and dosage.
This active-assistive robotic therapy tool is designed as a supplement to UE neuromuscular re-education, providing gravity-elimination and robotic active-assistance to the shoulder complex. BURT provides three anatomical planes of movement to the shoulder, and you can grade UE exercise across the motor learning spectrum, from PROM to AAROM and AROM.
BURT is highly adaptable, can be set up in less than five minutes, and can be easily moved to accommodate the patient. BURT includes a gaming system that motivates patients and is highly customizable; it supports therapist task-grading with features such as easy modification of cognitive stimuli, set and therapist-controlled initiation of robotic assistance, vibration, and visual feedback. BURT also aids in training normative functional movement patterns in the presence of reflexive synergies, by setting the precise isolation of a desired functional movement.
BURT isn’t just for treatment of stroke. The device provides UE ROM and repetitive motor training, which therapists can use to treat other diagnoses such as traumatic brain injury, spinal cord injury, and Parkinson’s disease.

The InMotion ARM and Motorika ReoGo
The ARM and Motorika ReoGo are end-effector robots for the shoulder and elbow. The former is a well-studied clinical edition of the original MIT-Manus robot, which performs robotic assistance and resistance in two anatomical planes of movement. Additional hand and wrist attachments are available.
The Motorika ReoGo has a large distal point—one that resembles a joystick—upon which the patient’s hand rests. The device applies active or passive assistance to the shoulder complex. (Hand and wrist attachments are available.)

Tyromotion Diego
The Tyromotion Diego is a cable-driven bilateral device for adults and children. It has harness support at the wrist and forearm, which provides active-assisted shoulder flexion and extension.
Exoskeletons
Exoskeletons include the Armeo Power and Armeo Spring. Upper extremity exoskeletons are large mechanical devices that encompass the arm and hand and have multiple, precise attachment points between the anatomical joints and kinematic structure [9].
The Armeo Power provides robotic assistance for patients with more densely impaired motor functions, and it offers many advanced, research-grade features. The Armeo Spring provides gravity elimination for volitional movement, with no robotic assistance.
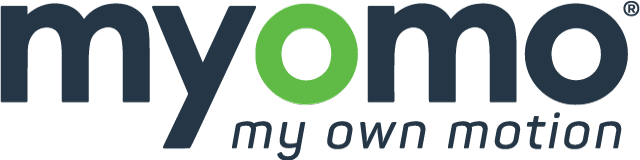
Portable assistive devices and orthoses
There are just a few robotic orthoses and portable devices on the market. The Hand of Hope and Tyromotion Amadeo are active-assistive robotic exoskeleton hands that attach to a workstation. The Hand Mentor Pro system is a distal semi-portable robotic device for the wrist and hand. The Myomo Myopro is a custom wearable myoelectric robotic orthosis for the elbow, wrist, and hand.
Rehabilitation trends are certainly changing.
Now that we know that cortical reorganization peaks in those first two weeks post-CVA, rehab interventions are adapting to increase intensity and dosage as quickly as possible. The movement toward earlier and more intensive task-repetitive training can put stress on therapists who are striving to balance patients’ needs with safety and other workplace demands.
Active-assistive robotic therapy can meet these needs by providing high-intensity treatment, without causing physical strain to therapists.These new technologies offer new opportunities for increasing patient engagement, improving patient outcomes, and providing a way to keep therapists healthy and injury-free in the process.
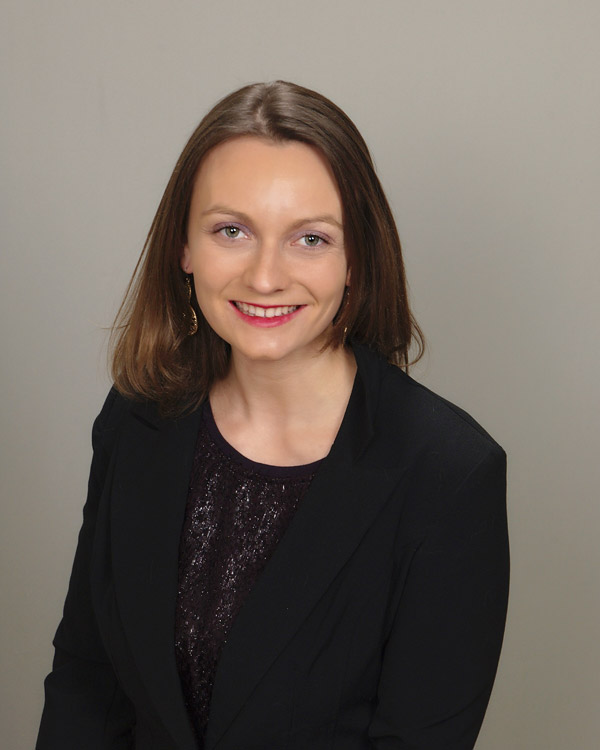
About the Author
Holly Mitchell, OTR, is clinical manager at Barrett Technology a Newton, Massachusetts-based manufacturer of the Barrett UE Robotic Trainer (BURT), an active-assistive robotic therapy system for the treatment of stroke and UE motor rehabilitation. She is an occupational therapist and member of AOTA with experience in the inpatient clinical rehabilitation setting. Holly has worked with neurological and orthopedic conditions and has additional training in stroke and prosthetic rehabilitation. She is an alumna of the Bay Path University Master of Occupational Therapy (MOT) program in Longmeadow, MA.
References
Krakauer, J. W., Carmichael, S. T., Corbett, D., & Wittenberg, G. F. (2012). Getting Neurorehabilitation Right – What Can We Learn From Animal Models? Neurorehabilitation and Neural Repair, 26(8), 923–931. http://doi.org/10.1177/1545968312440745
Kim, G., Lim, S., Kim, H., Lee, B., Seo, S., Cho, K., & Lee, W. (2017). Is robot-assisted therapy effective in upper extremity recovery in early stage stroke? —a systematic literature review. Journal of Physical Therapy Science, 29(6), 1108–1112. http://doi.org/10.1589/jpts.29.1108
Teasell, R., Foley, N., Salter, K., Bhogal, S., Jutai, J., & Speechley, M. (2016). Evidence-Based Review of Stroke Rehabilitation: Executive Summary, 17th Edition. Topics in Stroke Rehabilitation: 17th edition.
Saposnik, G., Teasell, R., Mamdani, M., Hall, J., McIlroy, W., & Cheung, D. (2010). Effectiveness of Virtual Reality Using Wii Gaming Technology in Stroke Rehabilitation: A Pilot Randomized Clinical Trial and Proof of Principle. Stroke, 41(7), 1477-1484. doi:10.1161/strokeaha.110.584979
Wolf, S. L., Thompson, P. A., Winstein, C. J., Miller, J. P., Blanton, S. R., Nichols-Larsen, D. S., … Sawaki, L. (2010). The EXCITE Stroke Trial: Comparing Early and Delayed Constraint-Induced Movement Therapy. Stroke, 41(10), 2309-2315. doi:10.1161/strokeaha.110.588723
Wagner, T. H., Lo, A. C., Peduzzi, P., Bravata, D. M., Huang, G. D., Krebs, H. I., … Guarino, P. D. (2011). An Economic Analysis of Robot-Assisted Therapy for Long-Term Upper-Limb Impairment After Stroke. Stroke, 42(9), 2630-2632. doi:10.1161/strokeaha.110.606442
Maciejasz, P., Eschweiler, J., Gerlach-Hahn, K., Jansen-Troy, A., & Leonhardt, S. (2014). A survey on robotic devices for upper limb rehabilitation. Journal of NeuroEngineering and Rehabilitation, 11(1), 3. doi:10.1186/1743-0003-11-3
Díaz, I., Gil, J. J., & Sánchez, E. (2011). Lower-Limb Robotic Rehabilitation: Literature Review and Challenges. Journal of Robotics, 2011, 1-11. doi:10.1155/2011/759764
Jarrassé, N., Proietti, T., Crocher, V., Robertson, J., Sahbani, A., Morel, G., & Roby-Brami, A. (2014). Robotic Exoskeletons: A Perspective for the Rehabilitation of Arm Coordination in Stroke Patients. Frontiers in Human Neuroscience, 8, 947. http://doi.org/10.3389/fnhum.2014.00947